Science
Perspective oxide epitaxy
The main challenges for the epitaxy of high-quality oxide layers are identified as the high oxidization potentials needed to achieve many desired compounds, the high temperatures required for numerous oxide phases to form, and the high temperatures necessary to grow films in adsorption-controlled growth modes. To overcome these challenges, various possibilities exist. Thermal laser epitaxy and CO2 laser heaters are deemed especially promising in this regard, because with this technology growth in extreme environments is possible.
This work was recently published in Applied Physics Letters Materials.
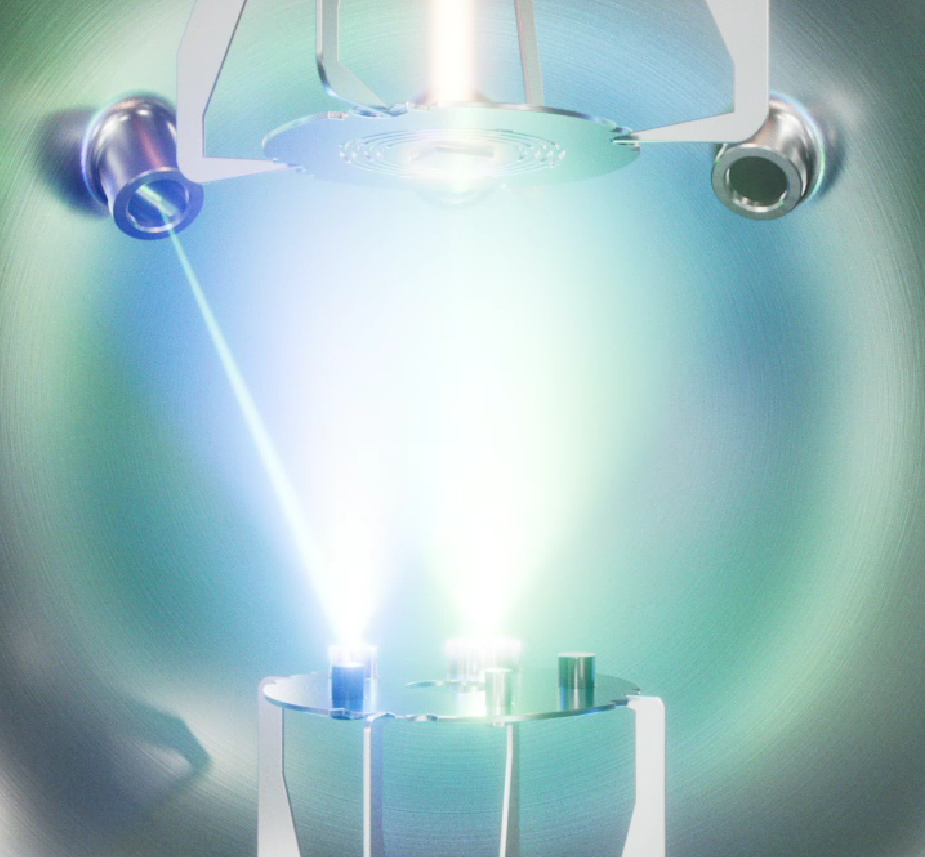
Sapphire Surfaces
Heating c-plane sapphire to a temperature of 1700 °C results in a perfect surface for subsequent epitaxy. These surfaces are characterized by atomically flat double-stepped terraces with widths exceeding 1 μm. The atoms on the surface rearrange in a singular in-plane orientation of the (√31x√31)R9° surface reconstruction, as shown in the reflection high-energy electron diffraction (RHEED) image on the right. The observation of up to 20 Laue circles indicates the high crystal quality of the surface.
This work was recently published in Advanced Materials.
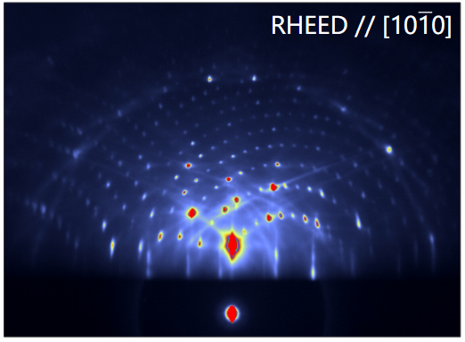
Evaporation of All Elements
We have explored the deposition of elemental metal films with TLE. So far we have succeeded in depositing films with thicknesses ranging from 1 to 500 nm on 2 inch Si wafers. All elemental sources could be evaporated in the same setup with growth rates between 0.01 and 1 Å/s by varying the laser power. Due to the inherent efficiency of the TLE process, significantly less power is required compared to high-temperature effusion cells and e-beam evaporators. A set of sample examples is shown below. The films are dense and homogeneous and have a very smooth surface morphology. These results show that laser evaporation is well-suited for the growth of complex compounds with excellent control.
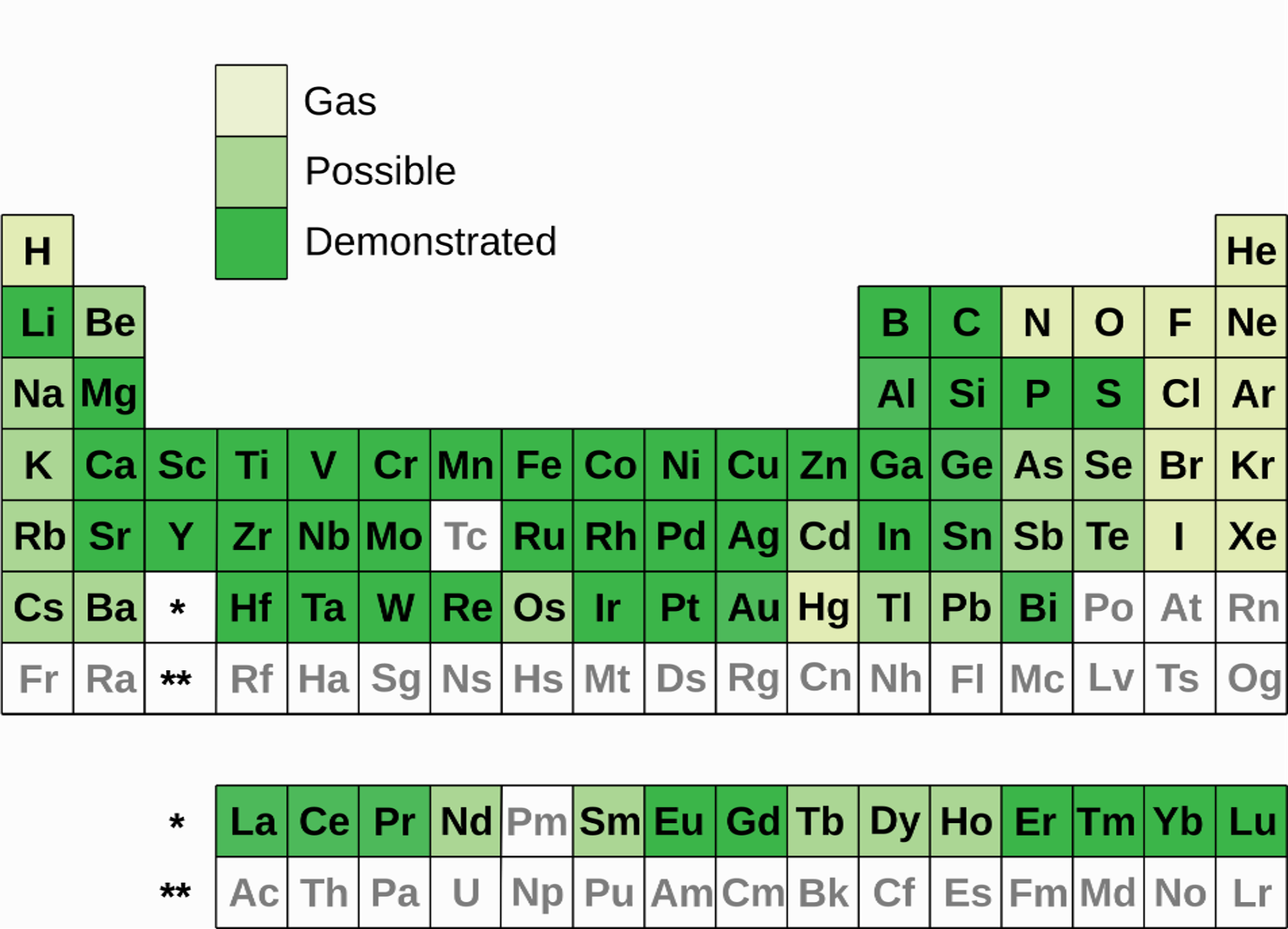
In situ surface preparation
Oxide substrate surfaces can be prepared simply by high-temperature annealing in the growth chamber. This removes the need for chemical treatments and ex situ annealing. The process takes only a few minutes. Specifically, the successful surface preparation of doped SrTiO3 (001), LaAlO3 (001), NdGaO3 (001), DyScO3 (110), TbScO3 (110), MgO (001), and Al2O3 (0001) surfaces is demonstrated.
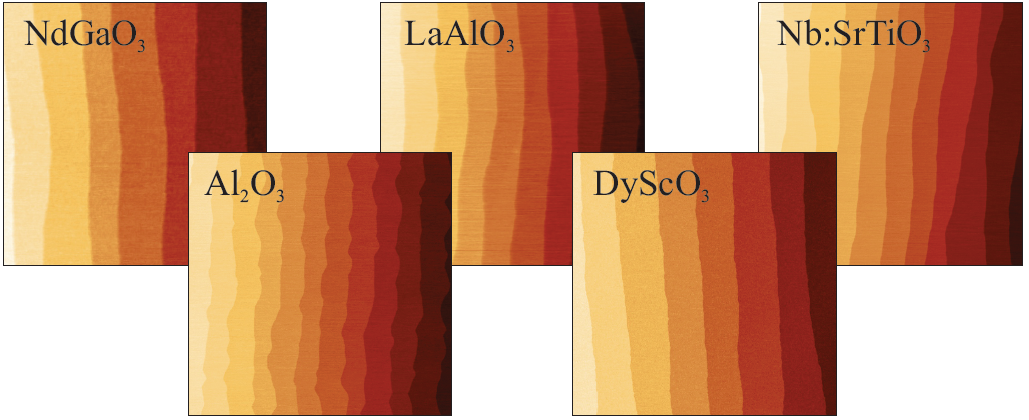
TLE publications
An overview of the key scientific publications about thermal laser epitaxy.
2019-05 / AIP Advances 9: Film deposition by thermal laser evaporation
2021-02 / Journal of Laser Applications 33: Thermal laser evaporation of elements from across the periodic table
2021-08 / Applied Physics Letters Materials 9: Thermal laser evaporation for the growth of oxide films
2021-08 / Journal of Vacuum Science & Technology A 39: Epitaxial film growth by thermal laser evaporation
2022-09 / Thin Solid Films 758: Ultrasmooth graphene-coated metal thin films on sapphire grown by thermal laser epitaxy
2022-12 / Journal of Applied Physics 132: Thermal laser evaporation of elemental metal sources in oxygen
2023-05 / Journal of Vacuum Science & Technology A 41: Why thermal laser epitaxy aluminum sources yield reproducible fluxes in oxidizing environments
2023-10 / Crystal Growth and Design 23: Thermal Laser Epitaxy of Carbon Films
2024-04 / Applied Physics Letters Materials. 12: State of the art, trends, and opportunities for oxide epitaxy
2024-07 / Journal of Vacuum Science and Technology A. 42: Growth of high-quality ruthenium films on sapphire
CO2 laser heating publications
An selection of publications enabled by direct substrate heating using a CO2 laser.
2018-03 / Applied Physics Letters 112: Independence of surface morphology and reconstruction during the thermal preparation of perovskite oxide surfaces
2020-03 / Applied Physics Letters Materials 8: In situ thermal preparation of oxide surfaces
2022-03 / Applied Physics Letters 120: Engineering the stoichiometry of a TiO2-rich SrTiO3(001) surface
2023-01 / Advanced Materials 35: Self-Assembly of Nanocrystalline Structures from Freestanding Oxide Membranes
2023-05 / Applied Physics Letters 122: Employing high-temperature-grown SrZrO3 buffer to enhance the electron mobility in La:BaSnO3-based heterostructures
2024-03 / Advanced Materials 23: Long-Range Atomic Order on Double-Stepped Al2O3(0001) Surfaces
2024-04 / Applied Physics Letters Materials. 12: State of the art, trends, and opportunities for oxide epitaxy
2024-06 / Advanced Materials 24: Interface Design beyond Epitaxy: Oxide Heterostructures Comprising Symmetry-Forbidden Interfaces